
Introduction
Acute lymphoblastic leukemia (ALL) is the most frequent malignant disease in childhood. Although cure rates have significantly improved over the last 30 years, therapy resistance of blasts and re-emergence of disease are still limiting problems in the treatment of 25% of patients.
At first presentation of childhood ALL, persistence of leukemic blasts during therapy is of crucial prognostic significance. In the multicentric ALL-BFM (Berlin-Frankfurt-Münster) study, all patients are uniformly treated during the first week of induction therapy. The principal therapeutic agent is the glucocorticoid (GC) prednisone, which is administered according to treatment response on days 1 through 7. The regimen also includes one dose of methotrexate, which is given intrathecally at day 1. Treatment response, assessed cytomorphologically after a 7-day induction pre-phase (“day 8 prednisone response”), provides one of the basic parameters for further treatment stratification. Patients with a reduction in peripheral blast count to less than 1000/µl (“good prednisone response”) have a more favourable prognosis than patients whose peripheral blast counts remain above 1000/µl (“poor prednisone response”).1 Differential therapy response is supposed to result from differential GC sensitivity of blast cells2, however, molecular mechanisms of GC action in vivo are largely unknown.
In recurrent disease, overall cure rates reach merely 40% and are particularly poor in certain entities. The probability of long-term survival after relapse can be predicted from a variety of well-established prognostic factors, the most important being the time of relapse (summarized in Henze and Stackelberg, 2002).3 Early recurrence of disease with respect to frontline therapy is associated with a high rate of non-response to treatment, shorter duration of second complete remission and a low event-free survival rate4. Furthermore, site of relapse and amount of residual leukemic cells during treatment (minimal residual disease - MRD) are highly significant, independent prognostic factors.4,5 Treatment of relapse usually implies intensified polychemotherapy containing high-dose elements. Although in most children second remissions can be induced, high risk patients require further treatment intensification by stem cell transplantation, which provides a better relapse-free survival rate than chemotherapy alone. However, it is also associated with higher treatment-related morbidity and mortality rates.3 Therefore, biological insights into molecular mechanisms determining treatment outcome and risk for subsequent relapse are particularly required for this group of patients to ultimately facilitate the development of alternative therapeutic approaches - including those following a rational design strategy.
Results
We aimed at identifying molecular determinants of poor therapy response and outcome at first presentation and at relapse of ALL to ultimately improve risk stratification and treatment strategies by i) characterizing leukemic blasts persisting during frontline induction therapy, ii) characterizing leukemic blasts at ALL relapse by gene expression profiling.
Leukemic blasts persisting during frontline induction therapy
To approach mechanisms of resistance to initial therapy, we addressed genome-wide gene expression in blasts persisting after one week of induction therapy (day 8) and their molecular signatures as compared with blast cells at initial diagnosis (day 0). In order to approach this issue experimentally, a procedure has been established including flow sorting of leukemic blasts by their leukemia-associated immunophenotype (figure 1A) and preparation of cRNA, starting from a small number of cells. This experimental approach facilitated investigation of patient samples with blast cell counts as low as 100 blast cells/µl.
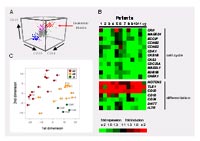
Blast cells from patients with precursor B-cell ALL were investigated using Affymetrix HG U133A microarrays, and genes commonly up- or down-regulated in blast cells under therapy were identified in matched pairs of day 8 and day 0 samples. In spite of the heterogeneous clinical features of the patients (mean rate of cytoreduction after 7 days of initial therapy = 82%, range between 33% and 99%), we were able to determine a set of 310 genes whose expression was commonly changed between day 8 and day 0 with an estimated false discovery rate of 0.05. The identified set of genes indicated inhibited cell cycling, and expression changes of multiple factors related to B-cell differentiation (figure 1B). These changes collectively suggested that gene expression in day 8 blasts is shifted towards resting mature B cells. To test this hypothesis, we isolated normal B cells from peripheral blood samples of leukemic patients and compared their gene expression to that of leukemic blasts using Principal Component Analysis. In a scatter plot using the first two principal components day 8 samples are positioned between day 0 samples and normal B-cell samples (figure 1C). Statistical significance of this observation could be established using the Jonckheere-Terpstra test. For both components, the changes in d8 blasts towards mature B cells were statistically significant (p = 0.0004 and 0.003). Changes of B-cell differentiation markers on protein level supported this finding.
Gene expression profiling of first relapse of ALL
To unravel molecular markers indicative of high risk of therapy failure and subsequent relapse, we performed gene expression profiling of 60 prospectively collected samples of ALL relapse patients enrolled in the current relapse trial of the BFM study group (ALL-REZ BFM 2002, http://www.kinderkrebsinfo.de/e1664/e1676/e1758/e5463/index_ger.html). Microarray analysis was performed on the Affymetrix platform using the HG U133A GeneChip. Evaluating our data set we first used published data from initial ALL6 to reliably predict genetic and immunophenotypic ALL subtypes in relapse samples, thus proving consistency of microarray-based classification across different stages of disease. Next, we tested several prognostic splits of relapse samples for differential gene expression using the regularized t-score7. Each split divided samples into two opposing groups according to a prognostic factor at ALL relapse. For splits "site of relapse" and "minimal residual disease - MRD" no significant differential expression of genes was detected. In contrast, relevant changes in gene expression were identified for "time of relapse" and a list of 83 genes mostly upregulated in very early compared to late relapse was obtained (false discovery rate 5%). Using Gene Ontology (GO) annotations of genes for statistical analysis we identified GO terms associated with cell cycle progression (i. e. S-phase and mitosis) to be significantly over-represented in this gene list (figure 2). Consistent with this expression pattern the cell cycle profile of samples from patients with very early relapse shows a significant increase in S-phase cells. These findings suggest that in very early recurrence of ALL cell cycle progression is significantly deregulated and that these defects in cell cycle progression might hamper the effectiveness of anti-proliferative chemotherapeutics.
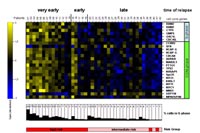
Outlook
In conclusion, genome-wide characterization of leukemic blasts persisting during frontline therapy and of leukemic cells re-emerging at relapse may have important clinical implications. The observed expression changes could differentially affect sensitivity towards agents used in the treatment of ALL. In particular, changes at the cell cycle level would have an impact on the anti-leukemic activity of genotoxic agents like daunorubicin and vincristine, which preferentially act against cycling cells. Identification of common and individual expression changes in future studies including enlarged patient cohorts may, therefore, contribute to develop individually tailored treatment programs in ALL.
Lit.: 1. Reiter A et al.: Chemotherapy in 998 unselected childhood acute lymphoblastic leukemia patients. Results and conclusions of the multicenter trial ALL-BFM 86. Blood. 1994 Nov 1; 84:3122-33. 2. Kofler R et al:. Resistance to glucocorticoid-induced apoptosis in lymphoblastic leukemia. J Endocrinol. 2003; 178:19-27. 3. Henze G and Stackelberg A: Treatment of relapsed childhood acute lymphoblastic leukemia. In: Treatment of acute leukemias. New directions for clinical research. Humana Press: Totowa, U.S.A., pp 199-219. 4. Gaynon PS et al.: Survival after relapse in childhood acute lymphoblastic leukemia: impact of site and time to first relapse--the Children's Cancer Group. Cancer 1989; 82:1387-95. 5. Eckert C et al.: Prognostic value of minimal residual disease in relapsed childhood acute lymphoblastic leukaemia. Lancet 2001; 9289:1239-41 6. Yeoh EJ at al.: Classification, subtype discovery, and prediction of outcome in pediatric acute lymphoblastic leukemia by gene expression profiling. Cancer Cell 2002; 1:133-43. 7. Tusher et al.: Significance analysis of microarrays applied to the ionizing radiation response. Proc Natl Acad Sci U S A 98; 9:5116.