
Introduction
Steroids control the differentiation and proliferation processes of cells and tissues. Recent data also suggest a participation of the steroid metabolism (Fig. 1) in the regulation of apoptosis, bone remodelling and neuroregene-ration. It is also not surprising that defects in steroid metabolism are contributing to the pathogenesis of many different multifactorial diseases like cancer (e.g. breast or prostate cancer), diabetes, diseases of cartilage and bone (e.g. atherosclerosis or osteoporosis) or neurological diseases (e.g. Alzheimer) (1,2). Monogenic mutations also cause severe diseases with loss of function of steroid pathway proteins (e.g. pseudohermaphroditism, D-bifunctio-nal protein deficiency) (3-6). As well hereditary diseases are known which are caused by disorders in biosynthesis of cholesterol (e.g. CHILD syndrome, Chondrodysplasia punctata, Smith-Lemli-Opitz syndrome and Zellweger syndrome) (7). Unfortunately, only few animal models for studies on steroid-related disorders are available yet. Identification and thorough analyses of more animal models are needed for better understanding of the molecular basis as well as the control of steroid metabolism, supporting the development of future medical treatment.
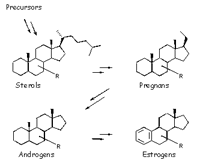
The steroid metabolism screen therefore focuses on the identification of new animal models for human steroid-related diseases. Since all diseases mentioned above are associated with the accumulation or disappearance of specific metabolites we intend to detect mutant mouse lines (knockout mice, ENU-mutated mice, transgenic mice) with altered steroid metabolism by looking for aberrations in the steroid concentrations in mouse plasma. Key steroids like cholesterol, 7-dehydrocholesterol, pregnenolone, or dehydro-epiandrosterone are extracted from the plasma and detected and quantified by gas chromatography/mass spectrometry (GC/MS). These results of will be related to the phenotypes of the mutants. Mice with dwarfism, cataracts, skin defects, malformations of limbs and distorted reproduction biology are the most interesting candidates for the screen since their phenotypic defects are also observed in human steroid-related diseases. The aim of the screen is the simultaneous extraction and detection of the steroids in one step instead of sequential steps, which is not performed elsewhere yet (8-10).
Results/Project Status
We are developing a screen for the analysis of key compounds of the steroid metabolism in mouse plasma. Blood is collected retro bulbar from 12 weeks old narcotised male mice and plasma is prepared by centrifugation and stored at -20°C. Plasma of female mice will be not analysed. Variations in steroid content due to estrous cycle would overlap changes caused by mutations. Plasma and not serum was chosen as the source for steroid assays to facilitate sharing of blood withdrawal with other GMC-groups. Plasma from mutant mouse lines is analysed in comparison to plasma of wild type animals. Since mutants are created in different wild type strains, biological variations have to be considered and the equivalent wild type animals have to be chosen for comparison. Steroids are extracted from the plasma either by organic solvents or preferably by solid phase extraction (SPE) on reversed phase columns (Fig. 2).
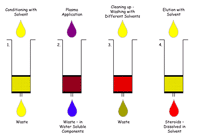
Detection and quantification is performed by gas chromato-graphy/mass spectrometry (GC/MS) (Fig. 3). The GC/MS-system used is the Agilent 5973N Mass Selective Detector (Fig. 5). The extracted steroids are separated on a gas chromatograph into fractions of relatively pure components and characterised on a mass spectrometer yielding qualitative and quantitative information (Fig. 4). As the GC/MS is used for steroid assays in many clinical chemistry studies we can refer to the experience of other research groups. The GC/MS-method is relatively cheap in establishment, assay performance, as well as maintenance.
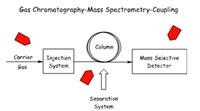
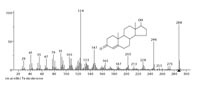
Steroid concentrations often are determined by EIA, ELISA, or RIA. These methods depend on the application of specific antibodies which should differentiate between various steroids. However, some cross-reactivity is known and for several steroid metabolites no antibodies are available. Running costs are as well quite high. Mass spectrometry is a method of choice here because of sensitivity, resolution, automation potential and low cost per sample.
As already said, the challenge of the screen is the simultaneous extraction and detection of key steroids in only one step. This is especially difficult because of the very low concentrations of steroids in blood and the very small amount of samples (50 µl in mouse, compared to 1 to 5 ml in men). The method for multiple steroid analysis is still in development. Up to now we identified the optimal GC-column and established a suitable method for the GC/MS-separation/detection of testosterone, pregnenolone, dehydro-epiandrosterone, methyltestosterone, cholesterol, noreth-androlone, 7-dehydrocholesterol and ergosterol. Currently, the LOD (limit of detection) of nonderivated steroids is 0.1 to 0.5 10-6 mol/l in the SIM-modus (single ion monitoring). Optimal steroid derivation lowering the LOD is as well established. Nevertheless, the low amounts of sample and the high coextraction of fatty acids are still the major problems. Further developments therefore aim to 1) lower the LOD and 2) gain higher purity and yield of the steroids after extraction. For this, we will use a new inert MS ion source to lower the LOD further. We consider iterative sample processing in cases where steroid concentrations are at extreme different levels, since only a single extraction of each plasma sample is possible. In such cases we intend to apply two or more different GC/MS-separation-detection protocols to the extracted steroid samples. Still, the main challenge is the material saving extraction of steroids without coextraction of fatty acids. The extraction method has to be optimised to take the different chemical properties of the steroids into account. Therefore we cannot use the same chromatographic separation as for the steroid analysis in men. We work on the removement of fatty acids using combined SPE-C18 cartridges and silica gel or ion exchange cartridges (tandem columns). A reliable quantification needs stable internal standards. As beeing more chemically stable than ergosterol, the new internal standard methyltesto-sterone is verified. To facilitate quality assessment and method development we participate in iterative consultations with other academic and industrial research groups.
Outlook
After finishing the method development we will provide a screen for the quantification of several steroids. This will be the primary screen of the German Mouse Clinic. Further we will establish a secondary screen for extended targeting of mouse lines, in which we found interesting features during the primary screen. Furthermore, we intend to detect new mouse models for steroid related diseases.
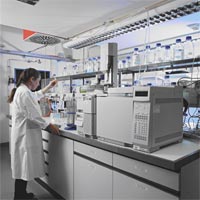
Lit.: 1. Valsamakis G et al. 11beta-hydroxysteroid dehydrogenase type 1 activity in lean and obese males with type 2 diabetes mellitus. J Clin Endocrinol Metab. 2004 Sep;89(9):4755-61. 2. Weill-Engerer S et al. In vitro metabolism of dehydroepiandrosterone (DHEA) to 7alpha-hydroxy-DHEA and Delta5-androstene-3beta,17beta-diol in specific regions of the aging brain from Alzheimer's and non-demented patients. Brain Res 969 (2003): 117-25. 3. Leenders F et al. Porcine 80-kDa protein reveals intrinsic 17 beta-hydroxysteroid dehydrogenase, fatty acyl-CoA-hydratase/dehydrogenase, and sterol transfer activities. J Biol Chem 271 (1996): 5438-5442. 4. Marijanovic Z et al. Closing the gap: Identification of human 3-ketosteroid reductase, the last unknown enzyme of mammalian cholesterol biosynthesis. Mol Endocrinol 17 (2003): 1715-1725. 5. van Grunsven E G et al. Peroxisomal D-hydroxyacyl-CoA dehydrogenase deficiency: Resolution of the enzyme defect and its molecular basis in bifunctional protein deficiency. Proc Natl Acad Sci U S A 95 (1998): 2128-2133. 6. van Grunsven E G et al. Peroxisomal Bifunctional Protein Deficiency Revisited: Resolution of Its True Enzymatic and Molecular Basis. Am J Hum Genet 64 (1999): 99-107. 7. Herman GE. Disorders of cholesterol biosynthesis: prototypic metabolic malformation syndromes. Hum Mol Genet. 2003 Apr 1;12 Spec No 1:R75-88. 8. Rommerts FF et al. Implications of progesterone metabolism in MA-10 cells for accurate measurement of the rate of steroidogenesis. Endocrinology. 2001 Dec;142(12):5236-42. 9. Shimada K, Mitamura K, Higashi T. Gas chromatography and high-performance liquid chromatography of natural steroids. J Chromatogr A. 2001 Nov 23;935(1-2):141-72. 10. Wolthers BG, Kraan GP. Clinical applications of gas chromatography and gas chromatography-mass spectrometry of steroids. J Chromatogr A. 1999 May 28;843(1-2):247-74.